By Frank Brueckner, Mirko Riede, Thomas Finaske, André Seidel, Steffen Nowotny, Christoph Leyens, Eckhard Beyer
Laser Metal Deposition (LMD) is used for repair/redesign as well as for manufacturing of new parts. Thereby, wire or powder filler material is reabsorbed in the laser-induced melt pool resulting in a strong metallurgical bond with the subjacent substrate in combination with a low dilution. Among various applications, LMD is an attractive process for jet engines to improve performance and efficiency as well as to contribute to more sustainability. In addition to design methods, such an improvement can be realized by lightweight structures and high-performance materials. Figure 1 shows the specific strength as a function of the temperature of high-performance materials. Since PMC structures are very important in the first stages of a jet engine, TiAl, Ni-base superalloys as well as CMCs are more relevant in hot engine areas.

High-Performance Materials
Corrosion and high-temperature resistant superalloys are state of the art in jet engines because of their ability to resist harsh conditions. Especially lightweight materials such as TiAl provide a significant improvement regarding structural strength, hardness and temperature capability due to the formation of an intermetallic compound. However, processing with these materials is a very demanding task and lamellar interface cracking easily occurs when typical cooling rates and temperature states in LMD are applied.
Even the direct deposition of a single layer onto a Ti-base substrate cannot be realized without cracking, see Figure 2.
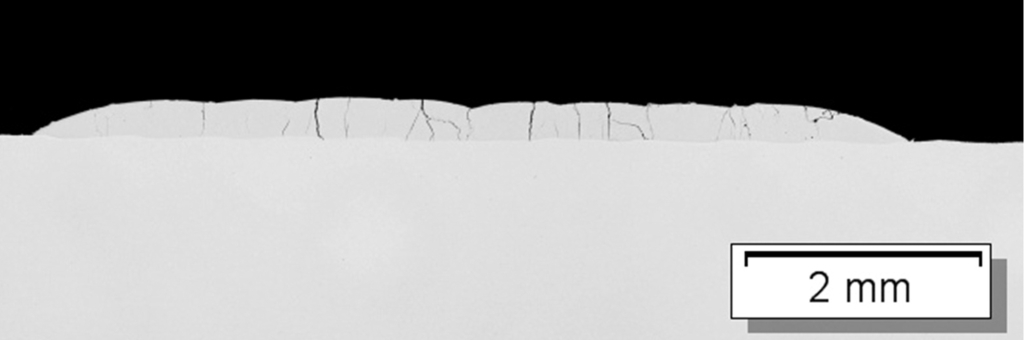
To overcome this problem, suitable temperature distribution; melt pool modifications; pre- and post-weld treatment and precise temperature regimes have to be considered while processing.
The latter was realized by an additional inductive substrate heating controlled by a closed loop camera system from IWS. A NIR temperature distribution of the substrate after the laser processing in a side view is shown in Figure 3.
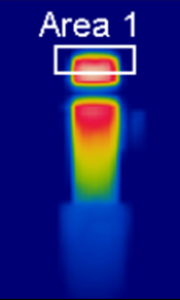
The ability to adjust temperature or even temperature profiles allows staying within the small process window of these materials. This leads to the crack-free deposition of single layers which can be accurately transferred to more complex multi-layer formations like the laser-generated TiAl blade in Figure 4.
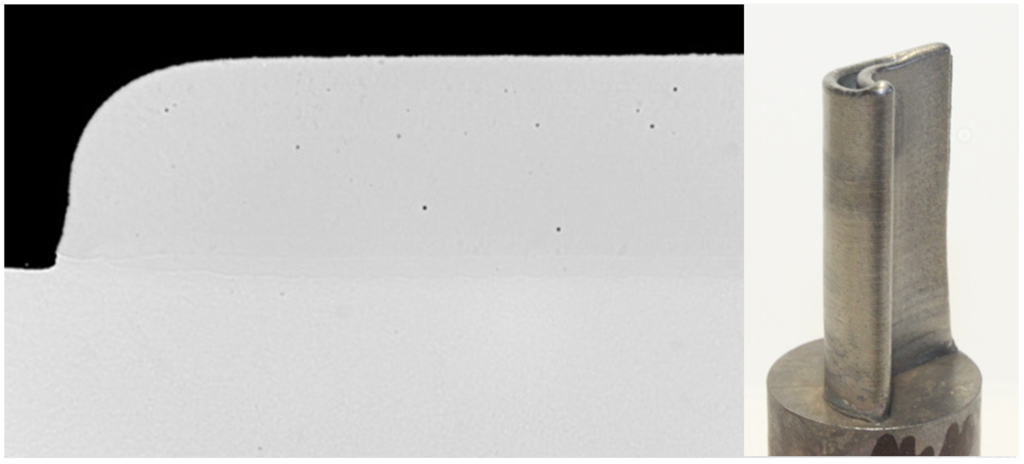
Metallographic investigations as well as computer tomography scans ensured that the deposition of the material was carried out without cracking or significant pores (see sample and cross-section in Figure 1). A porosity contents of below 0.01 percent was measured.
Lightweight Structures
As shown in Figure 1, there is an increased need for lightweight materials like carbon fiber reinforced plastics (CFRP) e.g., in cold sections of jet engines. Obviously, such composite structures requires proper bonding to the metallic components which are in many cases rather complex and not easy to handle (intelligent composite design) [2-4]. As a result of the strong differences of thermo-physical properties, especially of the melting/evaporation temperatures between metals and CFRP, adhesive bonds are often used. In most cases, the bonding is a multistage and time-/cost-consuming process. Hence, substantial research efforts are currently focused on the bonding of fiber laminates with metallic materials.
By means of a novel approach, a single-step process can be used to assure bonding over several layers of the CFRP (see Figure 5). Additionally, functional elements can be built directly on top of such bondings. Hence, the process can be divided into two phases.
Phase 1: At the beginning, the binder material is removed by the laser and subsequently infiltrated with a molten metal yielding a strong joint over several fiber layers of the CFRP.
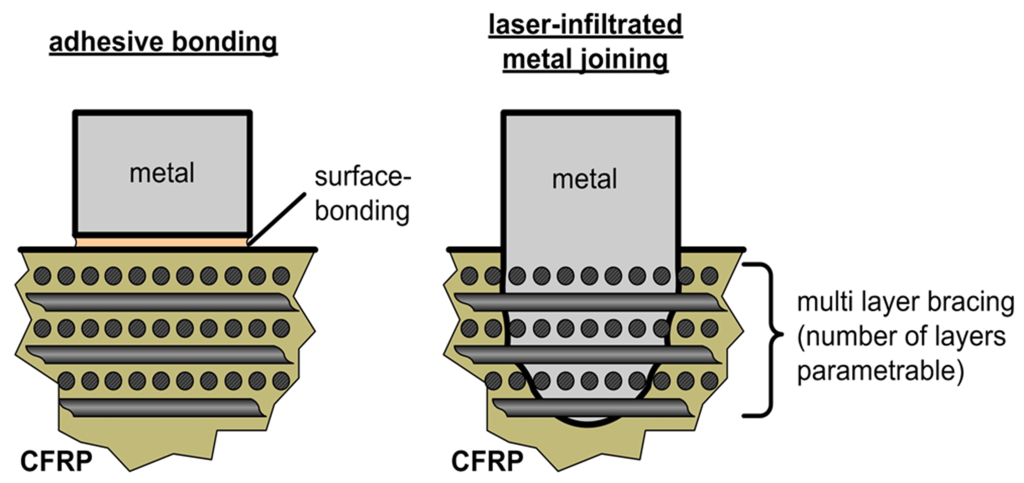
Phase 2: Based on the laser-infiltrated bond, functional elements can be built up without a transition zone to the infiltration metal (Figure 6, left) or with a gradient to the latter (see Figure 6, right).
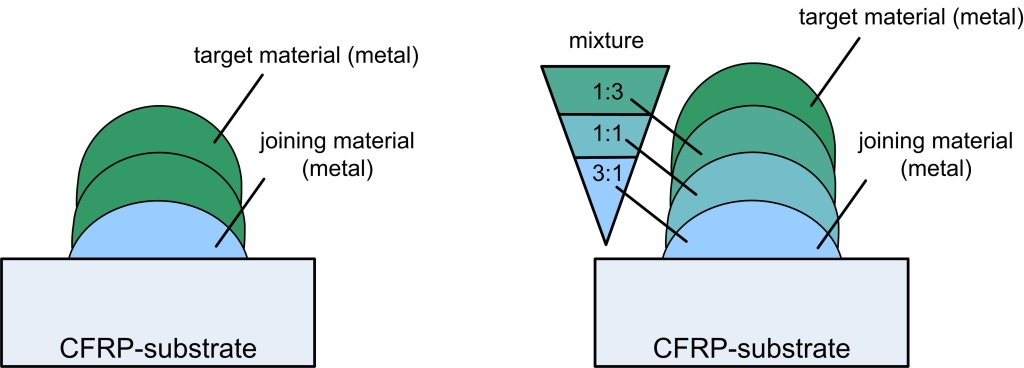
Experimental studies were carried out to produce high-strength metal-CFRP composite by laser-based metal-implantation (Figure 7a and 7b). As shown in Figure 7c, a high strength bond between a fiber reinforced plastic and light metal over several layers is feasible.
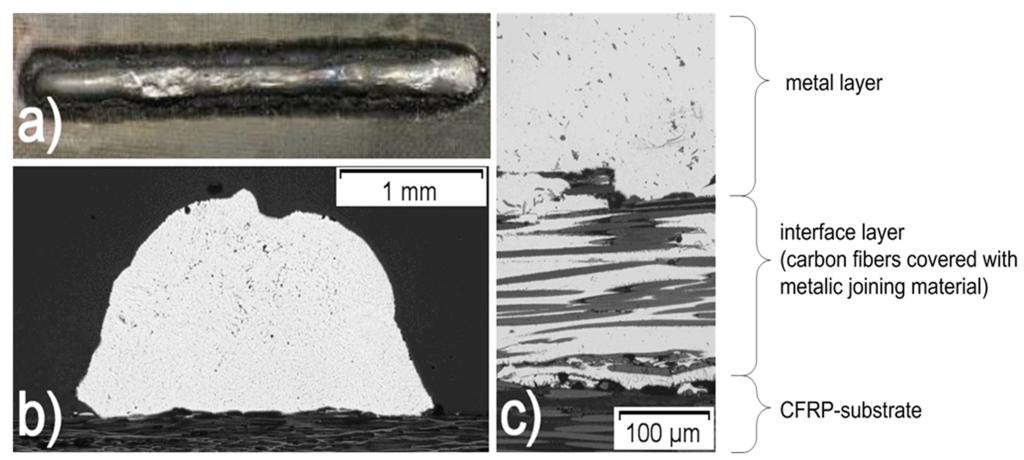
The described method of a precise laser-based adhesive bond and form fit could be used preferably for the following applications:
- The metal-CFRP joint may not be seen from outside as it is the case of rivet joints.
- The joint between the fiber laminate and the metal requires a strong local border, e.g., for mounting parts, edge protections or rotor blades.
- It might be especially relevant for large-area coatings on fiber laminates, e.g., lightning protection or design elements.
The authors of this article work for the Fraunhofer Institute for Material and Beam Technology in Dresden, Germany. Christoph Leyens and Eckhard Beyer are also associated with Technische Universität Dresden.
References
- Klocke F. Klink, A. Veselovac, D. Aspinwall, DK. Soo, SL. Schmidt, M. Schilp, J. Levy, G & Kruth, JP 2014, “Turbomachinery component manufacture by application of electrochemical, electro-physical and photonic processes” CIRP Annals – Manufacturing Technology, vol 63, no. 2, p. 703-726.
- Fecht: “Die CFK-Wertschöpfungskette im Griff,” VDI-Z Nachrichten154 Nr. 3.
- Drechsler: “Funktionsintegrierter Leichtbau,” Fachpräsentation FhG-Institut chemische Technologie ICT.
- Möller: “Novel method for joining CFRP to aluminium,” ScienceDirect Physics Procedia 5 (2010), p. 37.