By: Thomas Schopphoven, Fraunhofer Institute for Laser Technology ILT
Coatings for Wear and Corrosion Protection
When the integrity of a component is affected by the operational conditions, it may become necessary to additionally protect the material against this detrimental environment. Since most material damage processes, especially wear and corrosion, emanate from the surface or near surface regions, the application of coatings is the most suitable route to protect metallic surfaces. Coatings designed for the wear and corrosion protection must be an effective physical and chemical barrier, preventing the aggressive influence to the base material. The required thickness of the coating is dependent on the particular application and can range from a few up to several hundreds of micrometers. Generally, each coating system is aimed for specific functionalities, which among others include the chemical composition of the coating material, the adhesion to the base metal, the microstructure, the corrosion inhibition and the wear resistance.
While wear and corrosion protection coatings contribute to the performance, reliability and service life of many components across all industrial branches, these benefits often must be weighed against the environmental damage. For many years the most effective wear and corrosion protection systems were based on the use of chromate-rich surface treatments which turned out to potentially cause health problems for production workers and the community as a whole. The current legislation imposed by REACH (Registration, Evaluation, Authorization and Restriction of Chemicals) now prohibits the use of hexavalent chromium in almost all sectors. Similarly, this restriction applies to the use of nickel in electroplating, which has been classified as dangerous for the environment and toxic by the WHO (World Health Organization). As a result, REACH decisions have fostered the search for more advanced “green,” non-toxic and resource conscious coating technologies for the wear and corrosion protection. Many alternatives have been investigated, and so far the most promising technologies are thermal spray technologies, especially high velocity oxygen-fuel (HVOF) thermal spraying and laser material deposition (LMD).
The Search for “Green” Alternatives

(Diameter: 1.000 mm, Length: 10 m)
With HVOF thermal spraying, coatings out of a large range of materials can be applied, featuring a high wear resistance at relatively high deposition rates (1-4 kg/h). However, thermal spray coatings are technologically constrained in regards to the limited adhesion strength due to the poor mechanical bonding between coating and substrate. Moreover, thermal spray coatings are difficult to repair and often exhibit porosity levels in the range of 1-2%, lowering their corrosion resistance. Another disadvantage associated with HVOF thermal spraying is the comparatively high resource consumption of fuel, powder and oxygen. With LMD high-quality, almost 100% dense, pore- and crack-free coatings out of a wide variety of materials can be produced with a strong metallurgical bonding. Through a defined heat input and localized material deposition, LMD provides remarkable benefits over conventional welding processes and thermal spray technologies. Due to the very small heat input, a rapid solidification and a fine microstructure can be achieved with a property profile that meets, or even exceeds, the specifications of wrought or cast material. However, for the coating of large components, such as cylinders in the paper making industry (Figure 1), the LMD process is too slow (coating rate: 10-50cm2/min, deposition rate: < 0.5 kg/h). Furthermore, LMD layers are too large for many applications (>500 µm). In this layer thickness range, many conventional welding technologies, such as tungsten inert gas welding or plasma powder welding, offer cost advantages due to drastically lower investment costs. Various facilities and companies already addressed the reduction of processing times in LMD over the past years, by producing larger melt pools with higher laser powers in order to deposit more powder per time. In fact, the deposition rate could successfully be increased, but in turn the resource-consumption, as well as the heat input, was increased. The core issue—the simultaneous reduction of the layer thickness and coating time—could not be solved.
Ultra-high-speed LMD: The first economical coating process for thin, metallurgically bonded layers
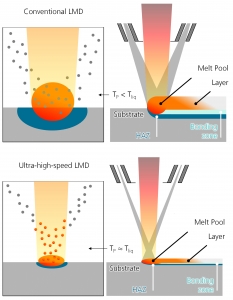
The maximum process speed as well as the minimum layer thickness in LMD is limited, because the heating and melting of the powder particles in the melt pool require a certain time and melt pool size. In Fig. 2 on the top, the process principle of LMD is schematically shown. Because the powder focus of conventional powder feeding nozzles is comparatively large (2-3 mm), most of the laser energy is transmitted to the substrate. As a result, most of the particles entering the melt pool have a considerably lower temperature Tp than the temperature of the liquid melt pool Tliq (Tp < Tliq). The general approach in ultra-high-speed LMD is to already heat the powder particles to melting temperature before they enter the melt pool (Tp ≈ Tliq), see Figure 2. To this, a larger amount of optical energy is deposited into the powder gas stream. At the same time, only a small fraction of transmitted energy is used to produce a thin melt pool on the surface of the substrate. Since both the necessary time and melt pool size are drastically reduced, a simultaneous increase of deposition speed by orders of magnitudes and the reduction of layer thicknesses to 10-250 µm can be achieved. Since less energy is deposited into the base material, only a very small heat affected zone (HAZ) and bonding zone with low dilution is formed. In order to deposit more energy into the powder gas stream, special powder nozzles are required, which produce a high optical density and a small powder focus diameter (0.5-1 mm).
Ultra-high-speed LMD for Internal Surfaces

For ultra-high-speed LMD on outside diameters, standard optics and can be used for the processing of internal surfaces, i.e., for the coating of cylinder sleeves, bearings and housings of the oil and gas industry or plastic and extrusion components. However, special processing heads are required. The optical components, the powder feed, the water cooling system and the tubes for the shielding gas have to be integrated into a compact housing. For conventional LMD various processing heads for different inside diameters and immersion depths have been developed and are used in industry. Due to the limited available space, up to now only multiple-jet coaxial or lateral powder feeding nozzles are used for internal LMD processing heads. However, the required small powder focus diameters for ultra-high-speed LMD can currently only be achieved with coaxial powder feeding nozzles with a continuous powder gas stream. Based on the basic principles continuous coaxial powder feeding concepts for the ultra-high-speed LMD on outside diameters, Fraunhofer ILT developed a novel continuous coaxial powder feeding nozzle and adapted it for an inside LMD processing head from IXUN Lasertechnik, see Figure 3.
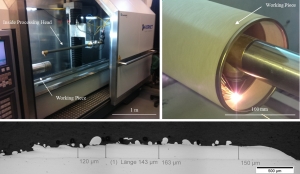
The inside processing head is integrated into a Hornet Laser Cladding high-speed LMD machine, see Figure 4, left. The system is based on a conventional lathe and retrofitted for ultra-high-speed LMD of rotationally symmetric components. With the system technology sound wear and corrosion protection layers in the thickness range of approximately 50-350 µm can successfully be deposited on the internal surface of cylindrical tubes with a minimal inside diameter of 100 mm with coating rates up to 200 cm2/min and deposition rates up to 1.5 kg/h, see Figure 4 on the right. On Figure 4 on the bottom a cross-section of a sound, metallurgically bonded ultra-high-speed LMD layer with minimal dilution and a thickness of approximately 100-150 µm is depicted.
Dipl.-Ing. Thomas Schopphoven is a Research Associate at Fraunhofer ILT and an expert for Ultra-high-speed Laser Material Deposition.