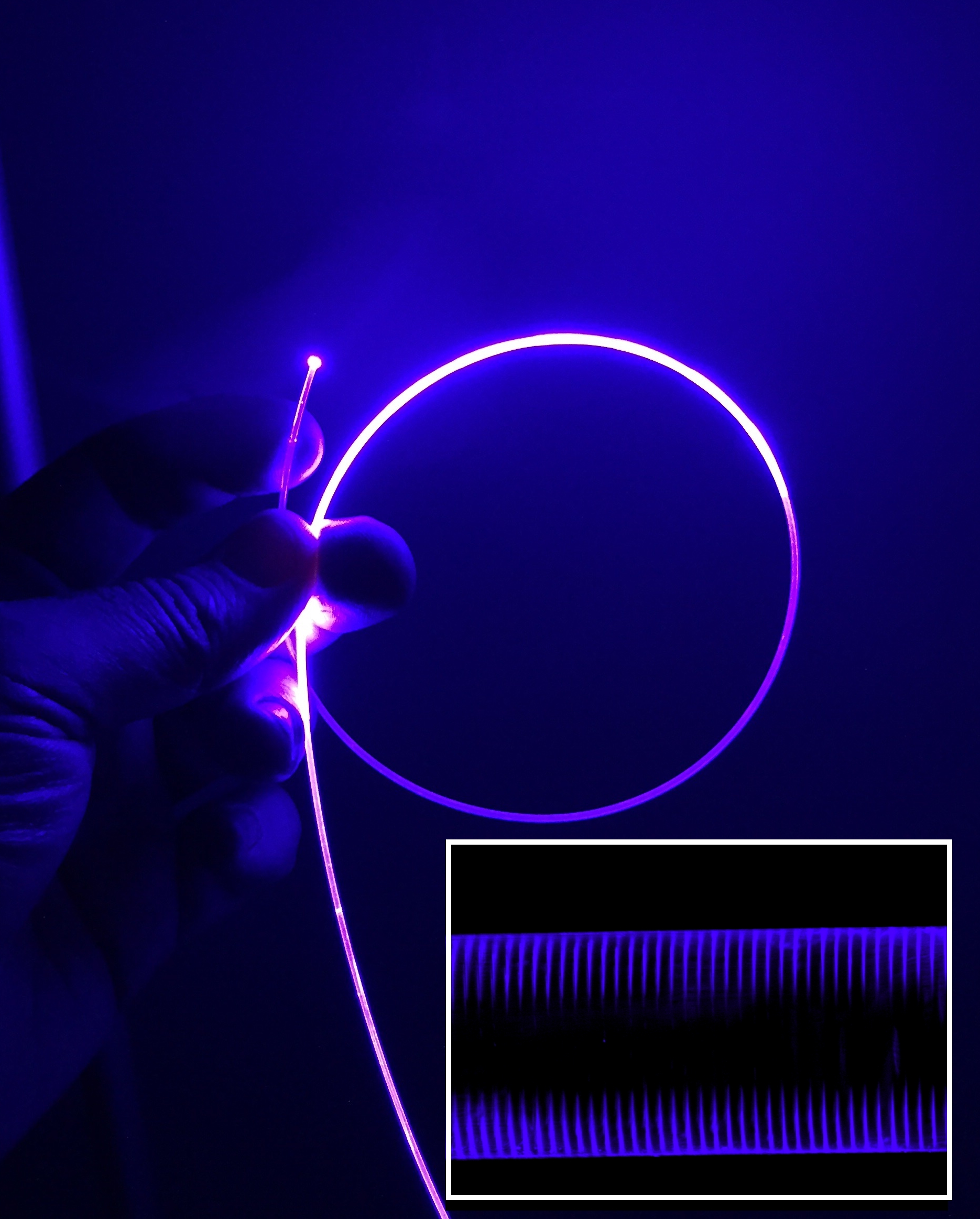
By Alan Conneely
Medical indwelling catheters used for vascular, respiratory and urological procedures, are implanted in the body for up to 3 months at a time and are therefore very susceptible to infection. In fact Catheter-associated urinary tract infection (CAUTI) is perhaps the most common device-associated infection in hospitals with over 1 million infections per year in the US leading to increased healthcare costs of >$400 m while causing significant patient discomfort and poorer medical outcomes.
A wide range of microbial pathogens are responsible for urinary infections with Escherichia coli and Staphylococcus aureus being the leading causes. Both the inner and outer surfaces of the catheter are susceptible to infection causing biofouling or encrustation of the catheter surfaces which typically ascends from the entry point (i.e., extracorporeal) to the bladder in the case of the Foley Urinary Catheter.
A multi-disciplinary project by the National Center for Laser Applications and the Bacterial Stress Response Group both at NUI Galway (Ireland) and Teleflex Medical (Reading, PA), which was part-funded by Enterprise Ireland, is aiming to develop an optic fiber delivered UV and Violet light based therapy to reduce the occurence of catheter associated infection.
Anti-microbial Impact of Light
UV-C light (200 nm to 280 nm) is widely used for disinfection purposes as the high photon energy directly breaks DNA bonds in microbes which leads to cell death. For the UV-A (315 nm to 400 nm) and Violet/Blue (400 nm to 495 nm) light range the light energy is not high enough to break chemical bonds but can interact with photosensitiser compounds, such as porphyrins, in the cell or local environment to generate Reactive Oxygen Species (ROS). High levels of ROS, such as hydroxyl radicals (·OH) or hydrogen peroxide (H2O2), are cytotoxic and lead to cell death by damaging the cell membrane, protein, DNA strands, etc. The use of UV-A and Violet light wavelengths is advantageous in this application as it is inherently safer than UV-C for human tissue and can be readily transmitted by optical fiber.

Side-emitting Optical Fibers
Optical fibers are designed to transfer light over large distances with minimal loss however optical fibers can also be specifically designed to promote leakage of light through the external surfaces. These side-emitting optical fibers (also known as light-diffusing or side-glowing fibers) are a useful device for remotely delivering light in medical applications such as photodynamic therapy. Numerous methods are available for producing side-emitting fibers:
- Microbending of the fiber axis as used in textiles
- Creating nanostructures in the fiber core by vapour deposition
- Mixing scattering microspheres or fluorescent additives into the fiber core or cladding material
- Modifying the geometry of the fiber and cladding to create asymmetries
- Increasing the refractive index of the fiber cladding material above that of the core material
- Selective removal of the cladding material by mechanical grinding or chemical etching
Most of these methods produce side-emitting fibers with a constant side-emission coefficient as a function of length therefore the light output reduces exponentially along the length of the fiber – this is not ideal for medical applications where a controlled irradiance is required. This issue may be counteracted by using a light source at each end of the fiber or by using an end-reflector however these solutions are not always technically feasible or cost effective. Another approach to achieve uniform or customized light intensity distribution is to modify the light scattering efficiency as a function of fiber length which is the approach taken by the use of laser machining in this project.

Laser Machining of Side-emitting Fiber
To create side-emission in the polymer optical fiber a UV Picosecond laser machining process is used to produce arrays of discrete micro-discontinuities in the optic fiber whereby each micro-discontinuity, consisting of an ablated pit and scattering filament that is <10 µm in diameter, generates some light leakage or side-emission. The easily reconfigurable nature of the laser scanning process means that the spatial density of the micro-discontinuities can be programmed so that the required side-emission coefficient as a function of fiber length can be achieved. In this study, 13 spatial density levels ranging from 1285 to 36000 micro-discontinuities per cm were used. Test fibers with side-emission lengths of 100 mm to 350 mm were produced – in the case of the 350 mm fiber there were 35 individual segments that were designed to produce a uniform light intensity profile along the length of the active region.
Light Coupling into Optical Fiber
Light Emitting Diodes (LEDs) are used as the test light source for coupling into the side-emitting fiber as this option provided the best combination of wavelength availability and output power. The highly divergent nature of the LEDs is challenging for efficient fiber optic coupling however a custom optical coupling system based on paired aspheric condenser lenses achieved up to 150 mW coupled into a 1 mm PMMA Polymer Optic Fiber for LEDs at 385 nm and 420 nm. This enabled the target irradiance or fluence rate of 3 mW/cm2 to be delivered for a 350 mm long side-emitting fiber.
Results & Future Plans for In Vitro Anti-microbial Testing
In the project to date anti-microbial studies have investigated the efficacy of direct exposure of 385 nm and 420 nm LED light for inactivation of E. coli attached to the silicone matrix used to make urinary catheters in a urine based media. Continuous irradiance with 385 nm and 420 nm light (3 mW/cm2) decreased cell counts to below detectable levels after 4 hours. These findings suggest 385 nm and 420 nm light as a promising antimicrobial technology for the prevention of biofilm formation on urethral catheters and future work will focus on the use of the side-emitting fibers for in vitro catheter testing.
Alan Conneely is Center Manager of the National Center for Laser Applications in NUI Galway, Ireland, a University-based laser processing research group. He can be reached at alan.conneely@nuigalway.ie.