By: Ying Liu, Wei Xiong, Lijia Jiang, Yunshen Zhou, Yongfeng Lu
Advanced three-dimensional (3D) micro/nanofabrication of functional devices represents a key research topic in modern nanoscience and technology and is critically important to numerous scientific and industrial applications. Among various existing 3D micro/nanofabrication methods, two-photon polymerization (TPP) based on laser direct writing is regarded as one of the most promising methods due to its unique combination of true three dimensionality and high spatial resolution.
The TPP technique is based on the nonlinear interaction of femtosecond laser pulses with photosensitive material, which induces a highly localized chemical reaction leading to polymerization of the photoresist with current resolutions down to 40 nm. The capability of the TPP technique is significantly determined by the properties of photoresists employed, which are electronic insulating in general. To increase the functionality and expand the applications of TPP, we used carbon nanotubes (CNT) as filling materials in the host polymers.

CNTs continue to deliver a huge impact on nanotechnology for their remarkable mechanical, electrical, thermal and optical properties. However, it is difficult to achieve both high CNT concentration and homogenous CNT dispersion due to the strong van der Waals interactions among individual CNTs. Moreover, the linear optical absorption of CNTs also limits the maximum doping level of CNTs in composite resins for nonlinear TPP lithography. The relatively low CNT loading concentration leads to limited performance of the composite resins. To overcome these limitations, a TPP-compatible composite material based on multi-walled carbon nanotubes (MWNT), thiol, and acrylic photoresist is presented in here. The schematic illustration of the composite preparation and 3D printing is shown in Fig. 1.
The TPP compatible composite polymer was prepared by directly mixing acrylic monomer, thiol, and photoinitiator with MWNT powder with various weight percentages. The resins prepared showed excellent dispersion of MWNTs through the composite resins and had a high stability last for one week under ambient conditions without obvious MWNT aggregation. Using TPP lithography, a fs laser beam was tightly focused into the composite resin to make 3D scans according to geometric user designs, resulting in solidified 3D micro/nanostructures with MWNTs simultaneously incorporated inside the polymer. After the TPP lithography, the samples were developed with the unsolidified resin rinsed away, leaving the 3D architectures of MWNT-based composite polymer on the substrates. A broad range of functional micro/nanostructures were fabricated, including micro-coil inductor, woodpile, spiral-like photonic crystal, micro-engine inlet fan, micro-car model and micro-gear, as shown in Fig. 2.

We also studied the distribution and alignment of MWNTs inside the polymer matrix. As shown in Fig. 3, precise assembly of MWNTs was achieved by the combination of TPP fabrication and direct pyrolysis. The length of MWNTs is longer than the laser focal volume, so the trapped MWNTs were forced to align with the laser scan direction. Volume shrinkage can cause tensile strength along the wires, which also contributes to the alignment.
By incorporating MWNTs into the acrylic polymer, the composite resin changed from an insulator to a conductor with greatly enhanced mechanical strength. With 0.2 wt% MWNT concentration, the electrical conductivity of the composite resin increased over 11 orders of magnitude and reached 46.8 S/m, as shown in Fig. 4(a). The superior conductivity of the MTA composite polymers originated from the high MWNT concentration and the uniform MWNT dispersion. Moreover, to utilize the alignment effect of MWNTs in composites, two bar-shaped channels were fabricated with two orthogonal laser scanning directions and showed a three-orders-of-magnitude difference in electrical conductance, which matched with the high anisotropy in electrical conductivity of MWNTs in directions parallel with or perpendicular to the MWNT axis.
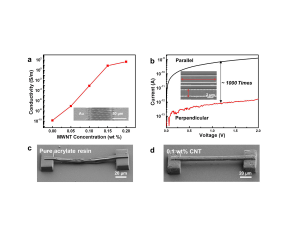
Two suspended microbridges in Fig. 4 (c, d) with the same design were fabricated using the acrylic and composite resins. Under the same fabrication condition, the bridge made by acrylic resin deformed seriously, while the one fabricated by the composite resin remained the straight shape without any obvious deformation, indicating the enhanced mechanical strength by the MWNT loading.
To demonstrate the potential of the composite resins, we fabricated a series of microelectronic devices, including arrays of capacitors (Fig. 5(a)) and resistors (Fig. 5(c)). Fig. 5(b) shows a typical hysteresis loop of a capacitor array containing 10 microcapacitors in parallel. Fig. 5(d) shows the frequency responses of a resistor array containing 20 zigzag microresistors in parallel. The impedance performance of the composite polymer transmission enables its application at high frequency range, such as RF electronics.

In summary, a TPP-compatible, homogenous composite resin with high MWNT concentrations has been developed. Various functional 3D micro/nanostructures using the composite resins have been successfully developed via the TPP lithography. Precise MWNT assembly of ~100 nm spatial resolution has been achieved by the combination of TPP lithography and thermal pyrolysis. The composites demonstrated to have increased mechanical strength and enhanced electrical conductivity. 3D printing of micro/nanostructures using highly conductive MWNT-based composites paves the way toward arbitrary precise assembly of MWNTs, which is promising for a broad range of device applications such as 3D electronics and MEMS/NEMS.
Ying Liu is a Ph.D student in Electrical and Computer Engineering at the University of Nebraska-Lincoln. Wei Xiong is a former UNL postdoctoral researcher and is now a professor at Huazhong University of Science and Technology (China). Lijia Jiang is a postdoctoral researcher in electrical and computer engineering at the University of Nebraska-Lincoln. Yunshen Zhou is a research associate professor of electrical and computer engineering at the University of Nebraska-Lincoln. Yongfeng Lu is Lott Distinguished University Professor of engineering at the University of Nebraska-Lincoln.