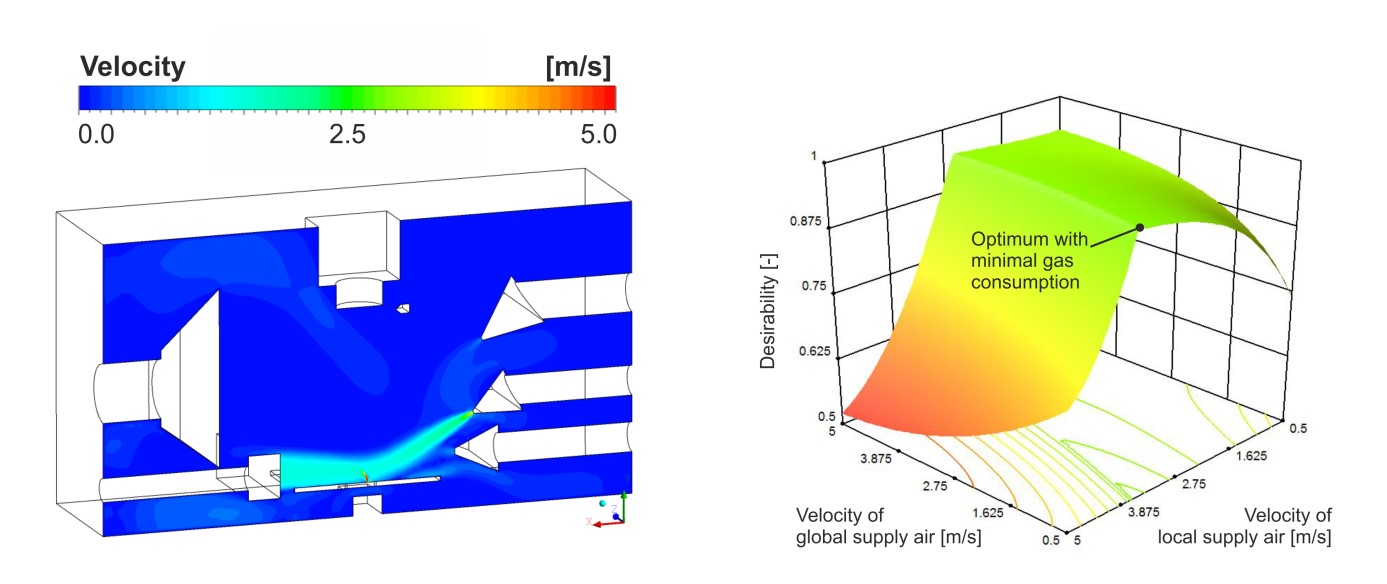
By Achim Mahrle1,2, Madlen Borkmann 2,1, Eckhard Beyer1,2, Michael Hustedt3, Christian Hennigs3, Alexander Brodeßer3, Jürgen Walter3, Stefan Kaierle3
1 Fraunhofer IWS Dresden, Germany
2 TU Dresden, Germany
3 Laser Zentrum Hannover e.V. (LZH), Germany
Developers and users of industrial remote laser beam welding applications are often faced with different challenges under the conditions of series production. First, those applications are preferably conducted without any localized gas shielding, and therefore, specific interactions between the laser radiation and the welding fumes are very likely to occur, causing an impairment of the process stability, the reliability and the weld seam quality. Second, welding fume residuals are capable of contaminating workpieces, optical components and other parts of the processing chamber, and they are also able to cause a serious pollution of the cabin atmosphere, because a significant part of the welding fume species is harmful or even toxic and carcinogenic. Each of these points gives a good reason to develop appropriate cabin air flow concepts, but in practice, it is still a challenge to design and optimize the air or gas flow because (i) the conditions of an ideal gas flow regime are uncertain, (ii) different gas flows are able to interact in complex manners, and (iii) it is costly to describe and monitor the gas flow characteristics inside the processing chamber experimentally. Consequently, a complementary combination of experimental and theoretical work has been performed to improve the understanding of inherent issues and relationships.
The experimental work was focused on the characterization of process phenomena and the determination of reliable welding conditions. For that purpose, a particular processing chamber was designed as shown in Figure 01. The interior view of this chamber shows inlet nozzles from a flat-jet type at different positions (1-3) on the right-hand side, as well as a global and a local exhaust air funnel (4-5) on the left-hand side. An additional cross-jet was applied to protect the laser optics (6). In this processing chamber, welding trials with a multi-mode fiber laser at an applied laser power of 3 kW and a welding speed of 2 m/min were performed on mild steel sheets with a thickness of 10 mm. Welds generated without any air flow showed no clear indications of a deep penetration process, and the weld depth was rather low. In contrast, the penetration was more than doubled under the influence of a well-defined gas flow. These findings emphasize the importance of an adapted cabin air flow with respect to the process efficiency. In the case of the investigations performed, local gas flow velocities in the range of 1 – 2 m/s above the weld zone were found to be sufficient to achieve this effect, and it was proven that larger values do not increase the penetration depth further on. In addition, it was found that a particular height of the welding plume is acceptable for stable welding regimes with maximum weld penetration depth. These processing conditions have been considered as a basis for optimization efforts regarding the cabin air flow.
However, with respect to the whole cabin flow, simple rules for an appropriate design are hardly available and optimal parameter configurations are difficult to find by means of empirical approaches because of the high number of control factors and factor combinations. To give an example, the individual air flow out of the applied flat-jet nozzle type is determined by 4 factors, namely the flow rate, the nozzle inclination, the distance to the processing zone and the outflow aperture. For the whole cabin air flow, 19 factors of influence have to be taken into account in total, which means that 219, i.e. more than a half million, factor-level combinations are possible if each factor is tested at only two value levels. Obviously, there is no alternative to Design-of-Experiments (DoE) methods which provide so-called screening designs to identify the most vital factors from a group of 19 factors with a minimal number of 192 runs. Such an analysis was performed by means of a Computational-Fluid-Dynamics (CFD) model to derive detailed information on cause-effect relationships regarding the cabin air flow. Exemplarily, Figure 02 (left) shows a computed air flow field for a particular parameter constellation. Process emissions were modeled as metal vapor inflow rate, and the height of a particular vapor concentration isoline was used as model response for the cabin flow evaluation. As a result of the screening analysis, 6 factors out of 19 were found as the most vital ones. With such a reduced number of factors, it became possible to apply a so-called multi-level Response-Surface-Method (RSM) as a basis for an air flow optimization. With a numerical effort of 157 additional computation runs, the functional dependencies between control factors and outcomes were quantified and described by a cubic regression model. Such a regression model is numerically easy to use and can be applied efficiently to determine optimal parameter configurations by computing the desirability function, plotted in Figure 02 (right) as a measure of the degree of fulfillment of defined optimization criteria, i.e. the limitation of the welding plume height to an acceptable level with minimal overall air or gas consumption.
The study has demonstrated a methodology to optimize the complex cabin air flow under the conditions of remote laser beam welding. However, the specific results cannot be generalized in a simple way as adaptable rules for the design of industrial processing cabins, because the characteristics of particular chambers, the spatial and temporal processing conditions, the type of applied air-flow components and the peculiarities of the specific welding applications always have to be taken into account for a profound analysis.
Acknowledgements
The work was performed in close collaboration by the Laser Zentrum Hannover e.V. (LZH) and the Fraunhofer IWS Dresden as part of the publicly funded research project “Steigerung von Prozessstabilität und Schweißnahtqualität beim Remote-Laserschweißen durch gezielte Strömungsführung mittels Anlagenadaption” (RemoStAad) with the reference number IGF 18149 BG. The authors acknowledge the financial and administrative support by the Bundesministerium für Wirtschaft und Energie (BMWi), the Arbeitsgemeinschaft industrieller Forschungsvereinigungen “Otto von Guericke e.V.” (AiF), the Forschungskuratorium Maschinenbau e.V. (FKM), and the Forschungsvereinigung Schweißen und verwandte Verfahren e.V. (DVS).
